Atmospheric Evolution and Habitability
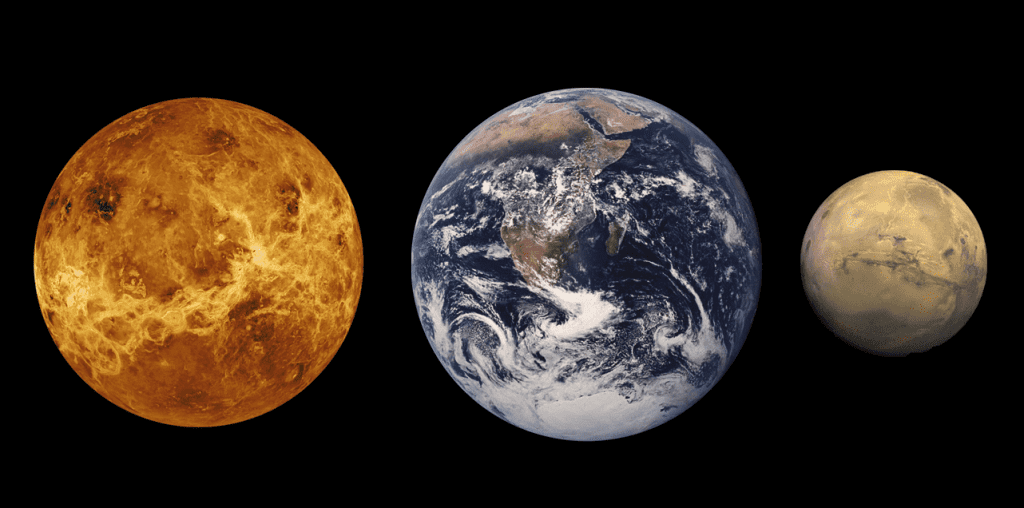
Our research group develops atmospheric models of varying complexity (1D, 2D, 3D) to simulate the atmospheres and habitability of the rocky worlds in our solar system (i.e., Venus, Earth, Mars, Titan) and assess their atmospheric/climatic evolution through time.
Dust Dynamics
We are studying the dynamics of dust particles in various solar system environments, including near the lunar surface, asteroid surface, ring systems, and in interplanetary space. This can include motion due to impacts and other disturbances or the motion of charged dust particles in plasma and electric fields.
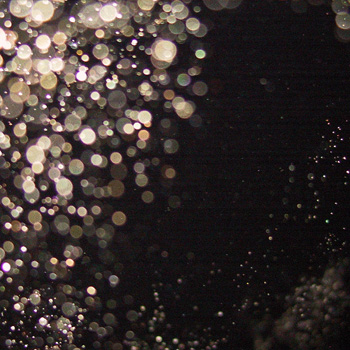
Exoplanets and Brown Dwarfs
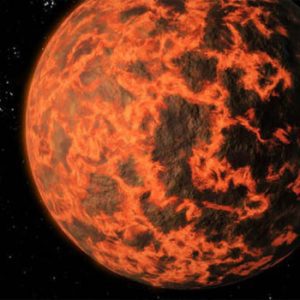
The Exoplanets Group uses space telescopes to reveal the sizes, temperatures, atmospheric properties, and orbits of many planets and brown dwarfs outside our solar system. We develop new theory and models to interpret and understand those data. We also lead several open-source software projects that provide tools for exoplanet research to the astronomical community.
Laboratory Astrophysics
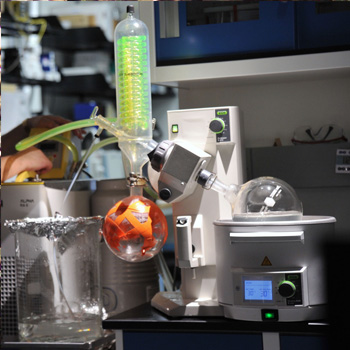
We trace the molecular basis for the evolution of interstellar clouds, the formation of Solar Systems, the incorporation of molecular species into planetary bodies, including comets and meteorites and how these systems evolve in time. Astrochemistry is often a culmination of different fields acting synergistically.
Laboratory Surface Science
We study the physical properties and compositions of the Moon, asteroids, and Mars. The surfaces of these bodies are covered with a fine-grained particulate regolith (or soil) that marks the boundary between internal geologic processes, and the space environment. What are these regoliths made of? How did they form and how have they evolved over time? Most important: what can this tell us about the earliest history of the solar system and the formation of planets?
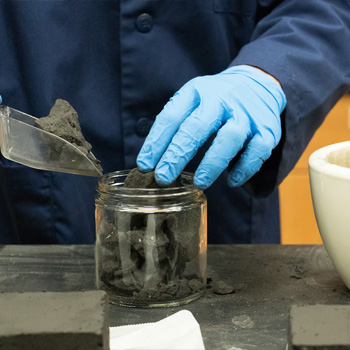
Microgravity & Planet Formation
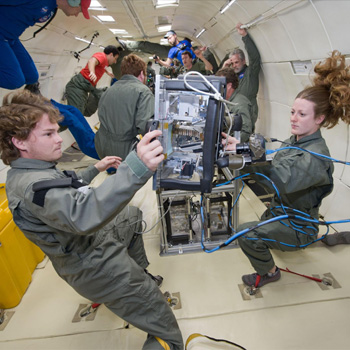
Simulations of planet formation that begin with planetesimals have reached a state where the main properties of the solar system are reproduced. Formation of planetesimals themselves remains poorly understood, however. The role of gravitational instabilities in the disk is not known. The outcome of interactions of small aggregates and dust in the presence of the protoplanetary nebula is also poorly understood.
Planetary Rings
We are studying the structure, composition, and evolution of Saturn’s rings through a combination of analysis of data from the Cassini Ultraviolet Imaging Spectrograph (UVIS) and numerical modeling of the rings. We combine multiple stellar occultations to understand the three-dimensional structure of the rings in the same way doctors use CT scans to study the structure of the body. These occultations provide exquisitely detailed maps of the finest structures in the rings which we can then compare to N-body simulations to better understand the dynamics of the rings and their origins.
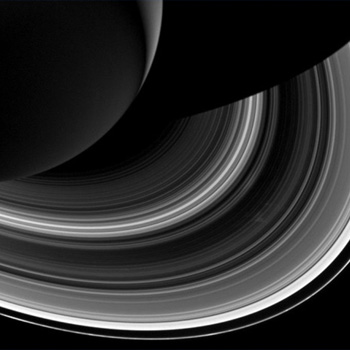
Small Bodies
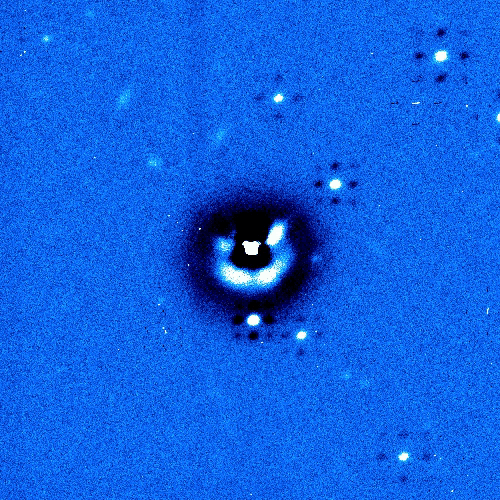
“Small bodies” are big on science! Small bodies are comets, asteroids, trans-Neptunian objects (TNOs), Trojans, and Centaurs that are all remnants from the planetary formation era 4 ½ billion years ago. Studying these objects’ behavior and evolution allows us to answer some of the most fundamental questions in astronomy and planetary science.